Pathways Methodology
Introduction
This page outlines the methodology behind the SCATTER Pathways tool and the key data sources and assumptions used. The Pathways Methodology is split into the following sections:
- What’s Changed? – Information on the updates that have been made in the 2023 updates to SCATTER since the first version available online.
- Target Analysis– Information on the sources and method for applying Net Zero and Science-based target comparison.
- How SCATTER Scenarios works? – Overarching method, starting point and key caveats of SCATTER pathways.
- Interventions – Methodology of each individual intervention including the ambition levels, key assumptions and data sources.
What’s changed?
The latest version of SCATTER Pathways, released in the summer of 2023, is now provided in an Excel-based tool to incorporate new functionality and provide improved options and flexibility to the tool. A summary of the key changes is provided below:
- Pre-defined scenarios – The previous online tool allowed users to select the level of ambition (usually 1 to 4) for 32 different interventions. This meant users could create their own bespoke pathways with different combinations of ambition levels across sectors. To simplify this, we have defined scenarios for the user in the latest version of the SCATTER Pathways tool. We have reviewed how the interventions have been used and the most commonly selected ambition levels to create 5 scenarios with pre-selected ambition levels. This still maintains the original principles of SCATTER and being able to see the results of varying levels of ambition while also providing the most typical or useful combinations. You can view the underlying ambition levels behind the pre-selected scenarios in the Pathways tool in the Results sheet. Please note, SCATTER was developed in 2017, some interventions have since been updated but others may reflect technologies and policies of that time.
- Target Comparison – In this latest development of SCATTER we have added in the ability to compare against two types of targets: a Net Zero and a Science-based target. This shows the user how close their selected scenario could get them towards these targets and their gap to target. The science-based target is based on the Grandfathered annual reduction rate estimated by the Tyndall Centre’s Local Authority carbon budget tool. More information on the methodologies is outlined below.
- Locally-set interventions – Based on feedback from Local Authorities, we have added in the option to set your own locally-defined ambition levels for the renewable energy interventions. This allows users more flexibility to define a target mix of renewables for the area which the tool will model alongside the other interventions. This means that users can now override the SCATTER assumptions for each of the renewable technologies modelled to your own local target or ambition and see the impact on your emissions pathway.
Target analysis
Tyndall Centre Carbon Budget Aligned Pathway
We have added in an indicative pathway which is aligned with The Tyndall Carbon Budget Tool. A Carbon Budget is defined as the allowed cumulative total of emissions over a period of years which ensures temperature change remains below dangerous levels [1]. This allows Local Authorities to understand the reductions required to stay aligned with the Paris Agreement and is often referred to as a science-based pathway. It is based on where a Local Authority needs to get to.
The carbon budgets used are based on analysis done by researchers at the Tyndall Centre for Climate Change Research at the University of Manchester in 2019. The budgets have a 2020 start year and are based on 2019 emissions data (the budget is from 2020 until 2100). They show an assessment of the remaining carbon budgets for UK local authorities for a 'well below 2C, pursuing 1.5C' climate outcome and use equity principles set out in [2] Anderson et al. (2020) and [3] Kuriakose et al. (2022). Please review the Tyndall Centre website for the full methodology.
Please note that the method applied by the Tyndall Centre is a CO2-only and energy-only budget whereas SCATTER includes other Greenhouse Gases and emissions beyond the energy system. This means that it is not possible to apply the total carbon budget to the SCATTER start point because they include different emission sources. The Tyndall approach also uses UK local authority greenhouse gas emissions national statistics as it’s start point which has methodology differences to SCATTER and can impact the total budget. Instead, the required annual reduction rate (from grandfathering allocation regime) has been taken and applied to the start point in SCATTER out to 2050. This serves to demonstrate the scale and speed of reductions required as opposed to the exact budget of emissions, in particular the need for urgent and large-scale reductions in the near future. It is recommended that the Tyndall-aligned pathway included in the Pathways Tool is used to assess whether the speed and scale of reductions in your selected scenario matches that of a science-based pathway. This analysis should be used alongside the Local Authority reports produced by Tyndall to assess whether you are staying within your carbon budget.
The Tyndall-aligned pathway is different to the SCATTER scenarios should be viewed as a top-down target that does not consider feasibility and the SCATTER scenario viewed as an interventions-based pathway which looks at the impact of different measures and what needs to happen.
[1] https://carbontracker.org/carbon-budgets-explained/
[2] Anderson, K., Broderick J., F., & Stoddard, I., (2020) A factor of two: how the mitigation plans of ‘climate progressive’ nations fall far short of Paris-compliant pathways, Climate Policy, 20:10, 1290-1304, DOI: 10.1080/14693062.2020.1728209
[3] Kuriakose, J., Jones, C., Anderson, K., McLachlan, C., and Broderick, J., What Does the Paris Climate Change Agreement Mean for Local Policy? Downscaling the Remaining Global Carbon Budget to Sub-National Areas (July 14, 2022). Renewable and Sustainable Energy Transition, http://dx.doi.org/10.2139/ssrn.4028406
Net Zero or Carbon Neutral targets
Users are also able to input their target year for carbon neutrality or achievement of Net Zero emissions for comparison against their modelled scenario. This takes the Local Authority starting point and applies a linear reduction to the target year. Emissions are presented at zero in the target year which assumes there is some level of removal or sequestration of residual emissions. This, however, is not modelled in the scenarios (e.g. SCATTER scenarios don’t include offsetting). Although not part of the tool, it is also recommended to have shorter-term interim targets as part of a Net Zero target.
How SCATTER scenarios work
The general method for calculating the emissions trajectories for the scenarios is based on factors for the change year-on-year in the local authority area in terms of the starting data point – for example fuel consumption, numbers of trees/animals, or levels of different types of waste.
The starting point for all the scenarios is the Inventory data. To find out your starting data, download your inventory and see the “Full table” tab which lists each emissions source. These emissions sources are referenced in the Interventions descriptions below.
There is one key area where this approach differs. For the energy supply baseline in the scenarios, national energy generation trajectories have been apportioned to local authorities (e.g. by land area) rather than using the actual reported data per area, to provide a better estimation of future capacity for the different Scenarios. Download this file to see your baseline electricity generation, and anticipated generation in 2030 and 2050 for each lever choice.
When multiple interventions are applied to an inventory area, the effect is the product of all these interventions.
Key caveats
As with any modelling, there are a number of assumptions that have been made to allow for projections and calculations to be made. For the assumptions related to individual interventions, please view the Interventions section. Some of the key caveats or elements most frequently asked about are listed below:
- Some interventions may not show any change to a new pathway if they are not applicable to your area or if there is insufficient baseline data available. Be aware that many of the interventions have interdependencies with others. Therefore, interventions should not be considered in isolation, and will not simply sum together.
- Pathways applies a calculated electricity factor based on renewable energy generated within the local boundary to the current year activity data, which is not applied in the calculation of your area’s inventory. Locally-generated electricity is prioritised, and the remaining demand is met with electricity imported from the national grid. The imported electricity follows the DESNZ (formerly BEIS) modelling for electricity emissions factors to 2100. This local electricity factor allows Pathways to provide a more accurate representation of the impact of local energy generation on emissions. As a result, your inventory figures may not exactly match the 2019 starting point data in your Pathways chart.
- Pathways incorporates emissions reduction measures as well as nature-based solutions for carbon sequestration. The tool does not include negative emissions technologies such as carbon capture and storage (CCS) as, due to the uncertainty in their developments, it is not currently feasible to model their future impact on emissions to 2050. For some emissions sources, the current suite of interventions does not forecast complete decarbonisation. As a result, the interventions in the tool will not allow a local authority to reach ‘net zero’ since there will always be some residual emissions, despite application of nature-based carbon sequestration. Options for offsetting residual emissions should be considered by local authorities outside of Pathways.
- Intervention options for heating and cooling technologies for both Domestic buildings and Commercial & institutional buildings are modelled as a range of technology mix scenarios, rather than an increasing ambition level. The 13 different technology mix scenarios for space heating and hot water are not listed in order of lowest to highest reduction impact on the overall trajectory, and therefore each scenario description should be read so that the most relevant scenario is applied.
Inputting your own data
Part of the new functionality allows user to input their own local renewable generation targets and the target year. This can be completed for all of the renewable electricity technologies currently in SCATTER but does not require users to specify small- or large-scale technology as in the SCATTER assumptions. The model is then able to use the input from the user to override the existing SCATTER assumptions and show the impact of these targets on the emissions trajectory. This has been set up to allow a combination of user input and SCATTER assumptions for renewables if needed or for certain technologies to be excluded all together. A linear trend in the supply is assumed from a 2019 starting point.
The supply is then matched against the demand at each year and the remaining demand is met by the national grid which factors in grid decarbonisation [1]. Whilst it is possible to input a value greater than your area’s total demand, this extra supply is assumed to be exported to the national grid or used elsewhere and so any further savings are not credited to the area. To support users, we have also allowed flexibility in the unit of the input so both generation (kWh) and capacity (kW) can be modelled. The conversion rate between the two is indicative only and not tailored for individual authorities. It uses a national average for generation for the renewable energy for the individual technologies. There is no further consideration of the degradation rates of the renewable technologies or further efficiencies because of newer technologies.
[1]https://www.gov.uk/government/publications/valuation-of-energy-use-and-greenhouse-gas-emissions-for-appraisal
Interventions
The methodology behind each intervention is outlined below and structured by sector:
- Agriculture and land use
- Domestic buildings
- Energy supply
- Commercial, Industrial and Institutional
- Transport
- Waste
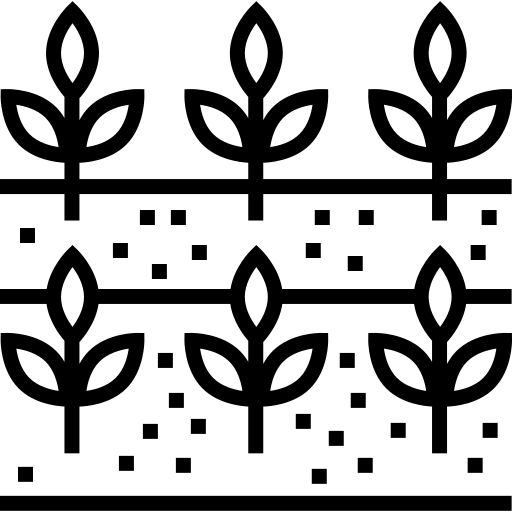
Agriculture and land use
Forestry
- Metric: Increase in forest land area
- Emissions sources affected: Emissions arising from land classified as “forestry”
- Interventions: Increase in forest land area
1. 5% increase in forest cover by 2030.
2. 10% increase in forest cover by 2030.
3. 16% increase in forest cover by 2030.
4. 24% increase in forest cover by 2030.
Original land use trajectories from DECC 2050 are used. Each land use type is mapped to a land use type used in the current SCATTER, by km^2. The rate of change in each land use trajectory is calculated for five-year chunks.
Land Management
- Metric: Increase in land used to grow crops for bioenergy
- Emissions sources affected: Emissions arising from land classified as grasslands, cropland, settlements and “other”.
- Interventions:
1. By 2050, 2% decrease in grassland. Cropland increases 5%. 13% increase in the coverage of settled land.
2. By 2050, 3% decrease in grassland, 1% decrease in cropland. 13% increase in the coverage of settled land as in BAU.
3. By 2050, 4% decrease in grassland and 1% decrease in cropland. 13% increase in the coverage of settled land as in BAU.
4. By 2050, 7% decrease in grassland. Cropland decreases 1%. 13% increase in the coverage of settled land as in BAU.
Original land use trajectories from DECC 2050 are used. Forestry is treated as a separate lever. Each land use type is mapped to a land use type used in the current SCATTER, by km2 The rate of change in each land use trajectory is calculated between 2020 and 2050. The mapping is as follows: Arable, for food crops (grades 1–3) LU_C Cropland Arable, for 1st gen energy crops (grades 1–3) LU_C Cropland Arable, for 2nd gen energy crops (grades 1–3) LU_C Cropland Grassland, for 2nd gen energy crops (grades 3–4) LU_G Grassland Grassland, for livestock and fallow (grades 3–5) LU_G Grassland Settlements LU_S Settlements Forests LU_F Forestland Other LU_O Other.
Livestock Management
- Metric: Number of livestock
- Emissions sources affected:
- Total number of dairy cattle
- Total number of non-dairy cattle
- Total number of sheep
- Total number of pigs
- Total number of horses
- Total number of poultry
- Interventions:
1. 0.2% annual growth in dairy cows & livestock.
2. No change from current levels.
3. 0.2% annual reduction in livestock numbers.
4. 0.5% annual reduction in livestock numbers.
Annual rates of change are applied for livestock. These are linear trajectories, but currently modelled in five-year periods. The trajectories are unchanged from the original DECC 2050 pathways and SCATTER V1. Trajectories impact dairy and non-dairy cattle, pigs, horses, and sheep, but not poultry.
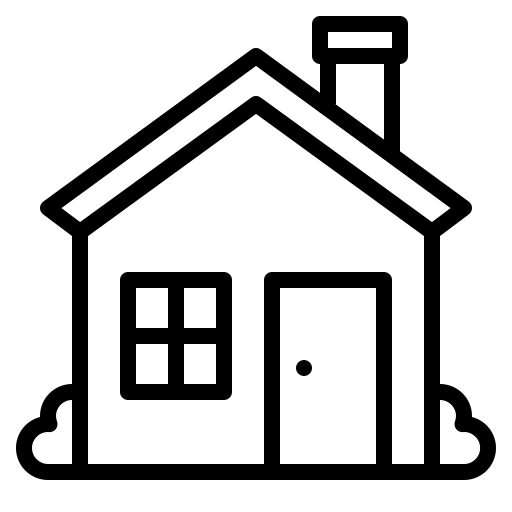
Domestic Buildings
Demand for heating and cooling
- Metric: TWh electricity and gas use by lighting, appliances and cooking
- Emissions sources affected:
- Domestic lighting, appliances, and cooking; Petroleum products (2)
- Domestic lighting, appliances, and cooking; Gas
- Domestic lighting, appliances, and cooking; Electricity
- Interventions:
1. Domestic lighting and appliance total energy demand decreases to 80% by 2050.
2. Domestic lighting and appliance total energy demand decreases to 66% by 2050.
3. Domestic lighting and appliance total energy demand decreases to 39% by 2050.
4. Domestic lighting and appliance total energy demand decreases to 27% by 2050.
Electrification of lighting, appliances, and cooking
- Metric: TWh electricity and gas use by lighting, appliances and cooking
- Emissions sources affected:
- Domestic lighting, appliances, and cooking; Petroleum products (2)
- Domestic lighting, appliances, and cooking; Gas
- Domestic lighting, appliances, and cooking; Electricity
- Interventions:
1. Small reductions in energy demand from cooking; no change in heat source.
2. Small reductions in efficiency of domestic cooking. Proportion of cooking which is electric increases to 100% in 2050.
This lever combines reductions in energy demand from domestic cooking with an anticipated shift to electrified heat. Scenario 1 assumes small efficiency gains but no shift in the share of domestic cooking which is electric; Scenario 2 increases electrification proportion to with 100% cooking electrified by 2050.
Domestic space heating and hot water – Demand
The key metric used in the demand trajectory in SCATTER is the total TWh energy consumed each year by households. Reductions in the total energy (TWh) consumed per household each year are applied to the total energy consumption for domestic water heating. This is the proportion of total energy reported domestic energy consumption for each fuel[1] allocated to hot water using statistics for Energy Consumption in the UK (ECUK)[2].
Total growth or reduction in demand per year is allocated to each fuel based on how much it is used in domestic water heating. The per-annum percentage changes in consumption of each fuel type for each intervention level are below.
Level 1 is an increase in domestic hot water demand, and level 2 assumes no change. These are proportionate to the scenarios mapped out in the original DECC 2050 Pathways calculator.
Intervention | Electricity | Solid hydrocarbons | Liquid hydrocarbons | Gaseous hydrocarbons |
1 | 0.102% | 0.007% | 0.018% | 0.245% |
2 | - | - | - | - |
3 | (0.072%) | (0.005%) | (0.013%) | (0.173%) |
4 | (0.171%) | (0.012%) | (0.031%) | (0.412%) |
Insulation of new houses
This metric is applied to the current heating demand for your local authority. Numbers of new houses are taken from local authority household projections for England[3], Northern Ireland[4], Scotland[5] and Wales[6]. Where these do not go to 2041, the data has been extrapolated based on the trend. This amounts to a 12% increase between 2020 and 2040 in the number of households across the UK, a 2-3% increase every five years.
Demolition rates are assumed to be 0.1%[7] of current housing stock, roughly 28,000 dwellings per annum.
- Emissions sources affected:
- Domestic space heating and hot water; Coal (2)
- Domestic space heating and hot water; Petroleum products (2)
- Domestic space heating and hot water; Gas
- Domestic space heating and hot water; Electricity
- Domestic space heating and hot water; Bioenergy & wastes
- Interventions:
1. All new houses are built to 2013 building regulations (no change).
2. From 2021, on average 50% of all new houses per year are built to 2013 building regulations; 40% to AECB standard; 10% to passivhaus standard.
3. From 2021, 30% of all new houses per year are built to 2013 building regulations; 40% to AECB standard; 30% to passivhaus standard.
4. From 2021, 100% new-build properties are built to passivhaus standard.
We have modelled interventions based on application of combination of the following standards to all new build properties:
- 2013 building regulations (base case)
- Association for Environment Conscious Building (AECB) standard
- The AECB standard refers to a standard developed by the Association for Environment Conscious Building, aimed at those wishing to create high-performance buildings using widely available technology at little or no extra cost.
- Passivhaus standard
- Passivhaus is an international energy performance standard. The core focus of Passivhaus is to dramatically reduce the requirement for space heating and cooling, whilst also creating excellent indoor comfort levels. This requires very high levels of insulation; extremely high performance windows with insulated frames; airtight building fabric; 'thermal bridge free' construction; and a mechanical ventilation system with highly efficient heat recovery. For more information see the UK Passive House Organisation website.
The key metric used in the insulation trajectory in SCATTER is the average kWh per year consumed by houses in the local area. To carry out these calculations, we partnered with the Association for Environment Conscious Building. Space heat demand has been modelled in PHPP (Passive House Planning Package).
The kWh/year energy consumption assumed for these standards, respectively, are:
| kWh/year |
New build 2013 building regulations | 10,335 |
New build AECB standard | 2,720 |
New build Passivhaus standard | 1,020 |
Comparison with EPC scoring (SAP)
The PHPP system has been used to estimate savings in space heat demand from buildings. This is a more accurate and detailed assessment method than the Standard Assessment Procedure (SAP), which is based on the annual energy costs for space heating, water heating, ventilation and lighting (minus savings from energy generation technologies) under standardised conditions, used for generating EPC scores. It uses a scale from 1 to 100. The method used means that the Specific Space Heat Demand of a building is often underestimated.
|
| PHPP Space heat demand for different housing | PHPP assessment of Specific Space Heat Demand | SAP assessment of Specific Space Heat Demand | SAP under (-ve) or over (+ve) estimate estimating SHD compared to PHPP |
|
| kWh/yr | kWh/m2.a | kWh/m2.a | % |
Bungalow | Original house | 15,275 | 230 | 161 | -30% |
| Deep IWI retrofit | 4,500 | 75 | 44 | -41% |
| Deep EWI retrofit | 3,142 | 51 | 32 | -37% |
Town house | Original house | 17,772 | 117 | 112 | -4% |
| Deep IWI retrofit | 5,183 | 40 | 42 | 5% |
| Deep EWI retrofit | 2,106 | 18 | 25 | 39% |
Semi-detatched | Original house | 11,714 | 179 | 140 | -22% |
| Deep IWI retrofit | 4,895 | 62 | 45 | -27% |
| Deep EWI retrofit | 2,507 | 26 | 22 | -15% |
Retrofit
The options presented allow you to change the proportion of houses that will receive different levels of retrofit assumed in your area in a target year of 2040.
Changes from SCATTER V1: We have changed the treatment of domestic housing to give a better idea of the anticipated activities sitting behind each reduction in total space heating demand.
The starting point for this is a weighted average of average kWh/year consumed by house types across England only – which has been applied to all local areas. A possible future improvement would be to localize this starting point per Local Authority, but this has not been done in this iteration as more localized and comparable data was not available. This average current per-house space heat demand starting point is comparable to that assumed in SCATTER V1.
The house types which have been modelled to generate this average, with the weightings, are:
- Bungalow (17%)
- 3-storey mid-terrace town house (35%)
- 2-storey semi-detached (48%)
The retrofit options are:
- Unimproved (repair & maintenance only)
- “medium” (deep inner wall insulation)
- “deep retrofit” (deep external wall insulation)
The assumed space heating demand (total kWh/household) are as follows:
|
|
| kWh/year |
House type | Original (unimproved) | Deep inner-wall insulation ("medium retrofit") | Deep external wall insulation ("deep retrofit") |
Bungalow | 15,275 | 4,500 | 3,142 |
Town house | 17,772 | 5,183 | 2,106 |
Semi-detached | 11,714 | 4,895 | 2,507 |
Weighted average | 14,444 | 4,927 | 2,478 |
- Interventions:
1. All current households remain at weighted average heat loss.
2. By 2050, 30% of current stock is retrofitted to a medium level; 20% deep retrofit.
3. By 2050, 40% of current stock is retrofitted to a medium level; 40% deep retrofit.
4. By 2050, 10% of current stock is retrofitted to a medium level; 80% deep retrofit.
Technology mix for heating
SCATTER considers eleven technologies for heating buildings:
- Gas boiler (old)
- Gas boiler (new)
- Resistive heating
- Oil-fired boiler
- Solid-fuel boiler
- Stirling engine μCHP
- Fuel-cell μCHP
- Air-source heat pump
- Ground-source heat pump
- Geothermal
- Community scale gas CHP
- Community scale solid-fuel CHP
- District heating from power stations
Trajectories are modelled as a linear trend from the current mix towards the selected end distribution in 2050. In order to estimate the current technology mix, we compared the scenarios defined in the DECC 2050 Calculator with the Energy Technologies Institute Clockwork model[8] results for Manchester.
The scenarios from the 2050 calculator have been organised into order for the trajectories by prioritising high electrification, and district heating, with dependence on solid fuel the lowest priority.
- Some scenarios have been excluded on the basis of their dependency on coal, and their similarity to other scenarios.
The primary fuel source, electrification level and heating system mix in 2050 for each scenario is summarised in the table below:
|
|
| Gas boiler (old) | Gas boiler (new) | Resistive heating | Oil-fired boiler | Solid-fuel boiler | Stirling engine μCHP | Fuel-cell μCHP | Air-source heat pump | Ground-source heat pump | Geothermal | Community scale gas CHP | Community scale solid-fuel CHP | District heating from power stations |
BASELINE (1) | Electrification level | Primary fuel source | 44% | 39% | 7% | 6% | 2% | - | - | 1% | - | - | 1% | - | - |
2 | Very low | Gas |
| 90% | 10% |
|
|
|
|
|
|
|
|
|
|
3 | Very low | District |
|
|
|
| 10% | 19% |
|
|
| 1% | 24% | 35% | 11% |
4 | Low | Gas |
|
| 10% |
|
|
| 90% |
|
|
|
|
|
|
5 | Low | Mixed / None |
|
|
|
| 5% |
| 16% |
| 25% | 1% | 23% | 23% | 7% |
6 | Low | District |
|
|
|
| 15% |
|
| 14% | 20% |
| 15% | 25% | 11% |
7 | Medium | Gas |
|
|
|
|
| 10% | 20% |
| 30% |
| 33% |
| 7% |
8 | Medium | Mixed / None |
|
|
|
| 10% |
|
| 25% | 25% |
| 13% | 20% | 7% |
9 | Medium | District |
|
|
|
|
|
|
| 58% | 30% | 1% |
|
| 11% |
10 | High | Solid |
|
|
|
|
|
|
| 50% | 30% |
|
| 20% |
|
11 | High | Gas |
| 20% |
|
|
|
|
| 60% | 20% |
|
|
|
|
12 | High | Mixed / None |
|
| 10% |
|
|
|
| 60% | 30% |
|
|
|
|
13 | High | District |
|
| 7% |
|
|
|
| 60% | 30% |
|
|
| 3% |
In order to translate these into year-on-year changes to the energy consumption reported at a local level in the DESNZ (formerly BEIS) fuel data, we calculated the proportion of space heating with each technology per year, applying technology efficiencies to understand the total demand for each fuel type. The change in demand in fuel each year is applied to the current demand. Technology efficiencies are summarised below:
| Heating / cooling efficiency (annual mean) |
Gas boiler (old) | 76% |
Gas boiler (new) | 91% |
Resistive heating | 100% |
Oil-fired boiler | 97% |
Solid-fuel boiler | 87% |
Stirling engine μCHP | 63% |
Fuel-cell μCHP | 45% |
Air-source heat pump | 200% |
Ground-source heat pump | 300% |
Geothermal | 85% |
Community scale gas CHP | 38% |
Community scale solid-fuel CHP | 57% |
District heating from power stations | 90% |
[1]Department for Energy Security & Net Zero, Sub-national total final energy consumption data; Total final energy consumption at regional and local authority level; Northern Ireland Utility Regulator; RETAIL MARKET MONITORING Annual Transparency Report For calendar year 2017
[2]Department for Energy Security & Net Zero, Energy Consumption in the UK (ECUK) 2018 Data Tables; Table 3.02: Domestic energy consumption by end use and fuel 1990 to 2017
[3]Office for National Statistics Household Projections for England, Table 406
[4]Northern Ireland Statistics and Research Agency, Northern Ireland Household Projections (2016 based), Summary
[5]National Records of Scotland, Household Projections for Scotland, 2016-based, Table 6
[6]StatsWales, 2014-based Household projections by local authority and year
[7] 2050 Calculator Tool (DECC) IX.A DOMESTIC SPACE HEATING AND HOT WATER http://2050-calculator-tool-wiki.decc.gov.uk/pages/31
[8] ETI (2015), UK Energy Systems Model Clockwork and Patchwork Results Charts http://www.eti.co.uk/programmes/strategy/esme
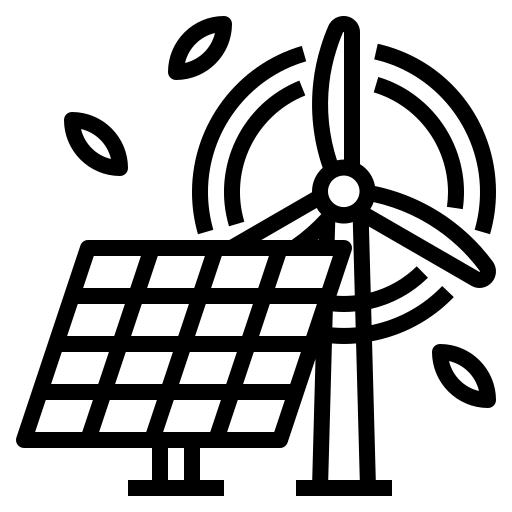
Energy Supply
The following interventions and assumptions apply when users do not override the renewable energy metrics with their own data in the Pathways Tool. Please see the section on Inputting your own data for more information on how his works.
Biomass/coal power stations
- Metric: TWh generation
- Emissions sources affected: fossil fuel generation and biomass generation recorded at a national level in DUKES.
- Natural Gas_pathways
- Gas Oil_pathways
- Coal_pathways
- Biomass Wood logs_pathways
- Biomass Grass/Straw_pathways
- Diesel_pathways
- Interventions:
1. No change in solid fuel power generation.
2. Solid biomass generation increases by 50% in 2025, dropping off after that; Coal phase-out follows trajectories from the National Grid's Two Degrees scenario.
3. Solid biomass generation doubles in 2025, dropping off after that; Coal phase-out follows trajectories from the National Grid's Two Degrees scenario.
4. Solid biomass generation quadruples in 2025, dropping off after that; Coal phase-out follows trajectories from the National Grid's Two Degrees scenario.
Biomass generation replaces fossil fuel powered generation. Trajectories for phase-out are taken from the National Grid Future Energy Scenarios[1] Two Degrees scenario.
Hydroelectric power stations
- Metric: TWh generation
- Emissions sources affected: Hydro, Hydro pumped storage
- Interventions:
1. By 2050, hydroelectric power generation increases by 12%.
2. By 2050, hydroelectric power generation increases by 20%.
3. By 2030, hydroelectric power generation increases by 40%, remaining fairly constant to 2050.
4. By 2030, hydroelectric power generation increases by 90%; by 2050 generation is 230% of current levels.
Increasing baseline hydroelectric power generation capacity. The TWh generated per GW capacity is calculated using the assumptions in the National Grid's Two Degrees scenario (2019).
Offshore wind
- Metric: TWh generation
- Emissions sources affected: Offshore wind
- Interventions:
1. Offshore wind triples by 2030, and increases steadily to 2050.
2. Offshore wind quadruples by 2030, increasing to five times current levels by 2050.
3. By 2030, offshore wind electricity generation is five times bigger than current levels, remaining relatively constant to 2050.
4. By 2030, offshore wind electricity generation is six times bigger than current levels, dropping slightly by 2050.
Increasing the rate at which offshore wind generation capacity changes. The TWh generated per GW capacity is calculated using the assumptions in the National Grid's Two Degrees scenario (2019).
Onshore wind
- Metric: TWh generation
- Emissions sources affected: Onshore wind
- Interventions:
1. Onshore wind grows by 50% by 2030, doubling by 2050.
2. Onshore wind generation doubles by 2030, increasing slightly to 2050.
3. Onshore wind generation is 2.2 times bigger by 2030, and 2.5 times bigger by 2050.
4. Onshore wind generation is 2.4 times bigger by 2030, tripling by 2050.
This lever works to increase the rate in installed GW per annum for onshore wind. The TWh generated per GW capacity is calculated using the assumptions in the National Grid's Two Degrees scenario (2019).
Small-scale wind
- Metric: TWh generation
- Emissions sources affected: Onshore wind not from Major Power Producers
- Interventions:
1. No change to small-scale onshore wind.
2. Onshore wind generation doubles by 2030, increasing slightly to 2050.
3. Onshore wind generation is 2.2 times bigger by 2030, and 2.5 times bigger by 2050.
4. Onshore wind generation is 2.4 times bigger by 2030, tripling by 2050.
Total small-scale wind capacity is calculated in GW. The change each year is calculated for each five-year period of time. This change is applied to current reported small-scale wind.
Solar PV – Large
- Metric: TWh generation
- Emissions sources affected: Solar PV from Major Power Producers
- Interventions:
1. 180% increase in large-scale solar PV generation by 2030; 320% increase by 2050.
2. 290% increase in large-scale solar PV generation by 2030; 540% increase by 2050.
3. 370% increase in large-scale solar PV generation by 2030; 720% increase by 2050.
4. 610% increase in large-scale solar PV generation by 2030; 1250% increase by 2050.
Solar PV – Small
- Metric: TWh generation
- Emissions sources affected: Solar PV not from Major Power Producers
- Interventions:
1. 180% increase in small solar PV by 2030; 320% increase by 2050.
2. 290% increase in small solar PV by 2030; 540% increase by 2050.
3. 370% increase in small solar PV by 2030; 720% increase by 2050.
4. 610% increase in small solar PV by 2030; 1250% increase by 2050.
Total small-scale solar PV is calculated in TWh generated, based on defined rates of total installed capacity (GW). The TWh/GW capacity generation efficiencies from 2017 - 2050 are taken from the National Grid's Two Degrees scenario (2019) for large scale solar PV, but the year on year rates of change are applied to the domestic / small scale solar PV recorded.
Tidal and Wave
- Metric: TWh generation
- Emissions sources affected: Wave/Tidal
- Interventions:
1. No change to current levels.
2. For areas with wave / tidal power, 14-fold increase by 2030, 70-fold increase by 2050.
3. For areas with wave / tidal power, 100-fold increase by 2030, 700-fold increase by 2050.
4. For areas with wave / tidal power, 320-fold increase by 2030, 1300-fold increase by 2050.
Tidal and wave generation is a combined scenario incorporating wave generation, tidal stream and tidal range The TWh generated per GW capacity is calculated using the assumptions in the National Grid's Two Degrees scenario (2019).
[1] Future Energy Scenarios, National Grid ESO 2019. https://www.nationalgrideso.com/future-energy/future-energy-scenarios/documents
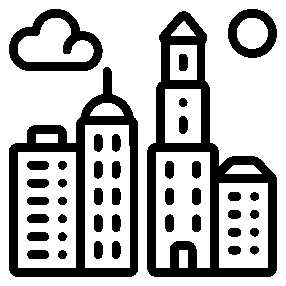
Commercial, Industrial and Institutional
Demand for heating and cooling
- Metric: Change in energy demand for commercial lighting, appliances and catering.
- Emissions sources affected:
- Commercial space heating, cooling, and hot water; Petroleum products (2)
- Commercial space heating, cooling, and hot water; Gas
- Commercial space heating, cooling, and hot water; Electricity
- Commercial space heating, cooling, and hot water; Coal (2)
- Institutional space heating, cooling, and hot water; Petroleum products (2)
- Institutional space heating, cooling, and hot water; Gas
- Institutional space heating, cooling, and hot water; Electricity
- Institutional space heating, cooling, and hot water; Coal (2)
- Interventions:
1. In 2050, commercial heating, cooling and hot water demand is 103% of current levels.
2. In 2050, commercial heating, cooling and hot water demand is 83% of current levels.
3. In 2050, commercial heating, cooling and hot water demand is 70% of current levels.
4. In 2050, commercial heating, cooling and hot water demand is 60% of current levels.
Changes are linear between 2020 and 2050.
Technology mix for heating and cooling
- Metric: Change in energy demand for commercial, industrial and institutional lighting, appliances and catering.
- Emissions sources affected:
- Commercial lighting, appliances, equipment, and catering; Petroleum products (2)
- Commercial lighting, appliances, equipment, and catering; Gas
- Commercial lighting, appliances, equipment, and catering; Electricity
- Commercial lighting, appliances, equipment, and catering; Coal (2)
- Institutional lighting, appliances, equipment, and catering; Petroleum products (2)
- Institutional lighting, appliances, equipment, and catering; Gas
- Institutional lighting, appliances, equipment, and catering; Electricity
- Institutional lighting, appliances, equipment, and catering; Coal (2)
- Institutional lighting, appliances, equipment, and catering; Petroleum products (2)
- Interventions - SCATTER considers eleven technologies for heating buildings:
- Gas boiler (old)
- Gas boiler (new)
- Resistive heating
- Oil-fired boiler
- Solid-fuel boiler
- Stirling engine μCHP
- Fuel-cell μCHP
- Air-source heat pump
- Ground-source heat pump
- Geothermal
- Community scale gas CHP
- Community scale solid-fuel CHP
- District heating from power stations
Trajectories are modelled as a linear trend from the current mix towards the selected end distribution in 2050. See Domestic Buildings for more detail on the modelling of these.
Energy demand for lighting, appliances and cooling
- Metric: TWh in energy demand for commercial, industrial and institutional lighting, appliances and catering
- Emissions sources affected:
- Commercial lighting, appliances, equipment, and catering; Petroleum products (2)
- Commercial lighting, appliances, equipment, and catering; Gas
- Commercial lighting, appliances, equipment, and catering; Electricity
- Institutional lighting, appliances, equipment, and catering; Petroleum products (2)
- Institutional lighting, appliances, equipment, and catering; Gas
- Institutional lighting, appliances, equipment, and catering; Electricity
- Interventions:
1. Commercial lighting & appliance energy demand increases 28% by 2050.
2. Commercial lighting & appliance energy demand increases 15% by 2050.
3. Commercial lighting & appliance energy demand decreases 4% by 2050.
4. Commercial lighting & appliance energy demand decreases 25% by 2050.
Total demand (TWh) from commercial, industrial, and institutional lighting and appliances increases in scenarios 1 and 2; decreases in scenarios 3 & 4.
Electrification of lighting, appliances, and catering
- Metric: Energy demand mix for commercial lighting, appliances and catering through electrification
- Emissions sources affected:
- Commercial lighting, appliances, equipment, and catering; Petroleum products (2)
- Commercial lighting, appliances, equipment, and catering; Gas
- Commercial lighting, appliances, equipment, and catering; Electricity
- Institutional lighting, appliances, equipment, and catering; Petroleum products (2)
- Institutional lighting, appliances, equipment, and catering; Gas
- Institutional lighting, appliances, equipment, and catering; Electricity
- Interventions:
1. Share of commercial cooking which is electric is at current levels.
2. By 2050, 100% of commercial cooking is electrified.
This lever combines reductions in energy demand from commercial cooking with an anticipated shift to electrified heat. Scenario 1 assumes small efficiency gains but no shift in the share of commercial cooking which is electric. Scenario 2 increases electrification proportion to with 100% cooking electrified by 2050. This results in an increase in electricity consumption and decrease in other fuels used for commercial cooking.
Industrial processes – Efficiency
- Metric: Total TWh consumption and energy mix from energy intensity of industry.
- Emissions sources affected:
- Industrial buildings & facilities; Petroleum products
- Industrial buildings & facilities; Gas
- Industrial buildings & facilities; Electricity
- Industrial buildings & facilities; Coal
- Interventions:
1. Industry moves to higher natural gas consumption, with electricity consumption falling before 2035 then remaining constant.
2. Industrial electricity consumption as a share of total energy increases between 2035 and 2050, reaching 40% of total energy consumption.
3. Industrial electricity consumption is 50% of total energy consumption by 2035; 65% by 2050.
4. Industrial electricity consumption is 50% of total energy consumption by 2035; 65% by 2050. Output falls by 2% every year for non-heavy industry.
This lever impacts the energy consumption trajectories from industrial buildings and facilities, and split by energy demand. The trajectories are focused on electrification of industry.
Industrial processes – Output
- Metric: GHG emissions from industrial processes
- Emissions sources affected:
- Iron and steel process emissions
- Non-ferrous metals process emissions
- Mineral products process emissions
- Chemicals process emissions
- Other industry process emissions
- Interventions:
1. Other industry process emissions are reduced at a rate of 2.6% per year.
2. Reductions in process emissions from all industry, with larger emissions reductions in the chemicals industry (0.4% pa) and other industry (2.6% pa). Metals and minerals industries also reduce process emissions 0.2% pa and 0.1% pa respectively.
3. Reductions in process emissions from all industry: general industry reduces process emissions at a rate of 4.5% per year. Chemicals emissions reduce 1% per year; metals 0.7% per year, and minerals 0.8% per year.
This lever impacts the process emissions from industrial activity. Separate trajectories are modelled for chemicals, metals, and minerals, industries. Growth rates are applied to the different industries' direct greenhouse gas emissions. Growth in "output index" from industry which applies to current process emissions and energy demand. Specific trajectories per industry type, mapped from 2015 - 2025 and 2025 – 2050.
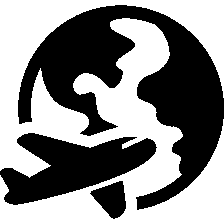
Transport
Domestic freight (road and waterways)
- Metric: TWh fuel use by on-road transport; TWh fuel use by waterborne freight
- Emissions sources affected: On-road transportation, waterborne transport
- Interventions:
1. By 2050, 47% increase in distance travelled by road freight; 40% increase in efficiency. In waterborne transportation, 15% decrease in use of waterborne transport.
2. By 2050, 27% increase in distance travelled by road freight; 60% increase in efficiency. In waterborne transportation, 6% increase in use of waterborne transport.
3. By 2050, 6% decrease in distance travelled by road freight; 71% increase in efficiency. In waterborne transportation, 25% increase in use of waterborne transport.
4. By 2050, 22% decrease in distance travelled by road freight; 75% increase in efficiency. In waterborne transportation, 28% increase in use of waterborne transport.
Domestic freight interventions affect both on-land and waterborne freight.
- On-land freight interventions are based on the on-road fuel consumption allocated to your Local Authority[1]. For this iteration of SCATTER, it has not been possible to separate the proportion of this attributable to freight. A UK-wide average has been applied to every Local Authority, based on the Local Authority specific data available for road transport fuel consumption[2].
- For Waterborne freight, total fuel consumption from national navigation increases as waterborne transport is increased.
Domestic passenger transport – Demand
- Metric: TWh fuel use across all transport
- Emissions sources affected:
- Petroleum products (2)Road transport
- Onroad Sc Petroleum
- Coal (2) Rail
- Petroleum products (2)Rail
- Interventions:
1. No change to total travel demand per person.
2. 5% reduction in total distance travelled per individual per year by 2030, staying constant at this level until 2050.
3. 15% reduction in total distance travelled per individual per year by 2030, staying constant at this level until 2050.
4. 25% reduction in total distance travelled per individual per year by 2030, staying constant at this level until 2050.
Domestic passenger transport - Modal Shift
- Metric: TWh fuel use by different transportation options
- Emissions sources affected:
- Petroleum products (2)Road transport
- Onroad Sc Petroleum
- Coal (2) Rail
- Petroleum products (2)Rail
The initial modal split used is taken from the National Travel Survey’s 2017/18 Average Distances Travelled by Mode[3]. The split represents the distribution between average distance travelled per transport mode in Urban Conurbations across England. “Urban conurbation” has been chosen with the intention of representing LA’s using the tool who have both urban and rural coverage. Full statistics are available summarized in the Factsheets published by the DfT[4]. The Rural Urban Classification is an Official Statistic and is used to distinguish rural and urban areas. The Classification defines areas as rural if they fall outside of settlements with more than 10,000 resident population[5]. The mode share data is a national breakdown of average mode share, which does not split by local authority, therefore this is not tailored to each local authority area.
The following changes are applied to reach level 4 ambition:
- % walking x3
- % cycling x3
- % using buses x3
- % using railways x1.5
- Levels 2 and 3 are mid-points between L1 and L4.
- Interventions:
1. No change to current national average modal split by total miles: 74% transportation by cars, vans and motorcycles.
2. Average modal share of cars, vans and motorbikes decreases from current national average 74% total miles to 56% in 2050.
3. Average modal share of cars, vans and motorbikes decreases from current national average 74% total miles to 47% in 2050.
4. Average modal share of cars, vans and motorbikes decreases from current national average 74% total miles to 38% in 2050.
Domestic passenger transport – Technology
- Metric: TWh fuel use by different transportation options
- Emissions sources affected:
- Petroleum products (2)Road transport
- Onroad Sc Petroleum
- Interventions:
1. Cars and buses are 100% electric by 2050. Slight increase in average train occupancy.
2. Cars and buses are 100% electric by 2040. Slight increase in average train occupancy and bus occupancy.
3. Cars and buses are 100% electric by 2035. Average occupancies increase to 18 people per bus km (from 12), 1.62 people per car-km (up from 1.56), and 0.42 people per rail-km (from 0.32).
4. Cars and buses are 100% electric by 2035. Average occupancies increase to 18 people per bus km (from 12), 1.65 people per car-km (up from 1.56), and 0.42 people per rail-km (from 0.32).
International aviation
- Metric: TWh fuel use from aviation
- Emissions sources affected:
- Aviation_fuel_Sc1
- Aviation_fuel_Sc3
- Interventions:
1. Department for Transport "Central" forecast for aviation. The "Central" forecast represents the DfT base-case. For reference see Pathways Methodology on the SCATTER website
3. Department for Transport "Low" forecast for aviation. The "Low" forecast encapsulates 'lower economic growth worldwide with restricted trade, coupled with higher oil prices and failure to agree a global carbon emissions trading scheme. For reference see Pathways Methodology on the SCATTER website.
Department for Transport growth forecasts[6] for international aviation, applied to both in-boundary airport emissions and to scope 3 emissions from people in the local area travelling. A yoy rate of change calculated between aviation in 2030, 2040 and 2050.
The "Central" forecast represents the DfT base-case; "Low" encapsulates 'lower economic growth worldwide with restricted trade, coupled with higher oil prices and failure to agree a global carbon emissions trading scheme'. A "High" scenario which projects 'Higher passenger demand from all world regions, lower operating costs and a global emissions trading scheme' is not included in the SCATTER model[7].
International shipping
- Metric: TWh fuel use by on-road transport; TWh fuel use by waterborne freight
- Emissions sources affected:
- Petroleum products (2)Road transport
- Onroad Sc Petroleum
- 004:Petroleum products_internal
- 004:Petroleum products_coastal
- Interventions:
1. By 2050, 48% increase in fuel use at UK ports.
2. By 2050, 18% increase in fuel use at UK ports.
3. By 2050, 2% decrease in fuel use at UK ports.
4. By 2050, 28% decrease in fuel use at UK ports.
For Waterborne shipping, total fuel consumption from national navigation increases as waterborne transport is increased. Road freight trajectories are developed from a combined impact of reduced distance travelled by HGVs (mostly diesel; electric trajectories only begin in the 2040s) with an increased efficiency (i.e. reduced energy demand per vehicle-km). The starting point for road freight efficiency is 5.29 TWh/bn vehicle-km (DESNZ (formerly BEIS) 2017), Road transport energy consumption at regional and local authority level, 2015) Baseline trajectory sees this efficiency increased to 3.15 TWh/bn vehicle-km by 2050. For the most ambitious (L4) scenario, the efficiency in 2050 is 1.34TWh/bn vehicle-km.
Road freight trajectories are developed from a combined impact of reduced distance travelled by HGVs (mostly diesel; electric trajectories only begin in the 2040s) with an increased efficiency (i.e. reduced energy demand per vehicle-km). The starting point for road freight efficiency is 5.29 TWh/bn vehicle-km (DESNZ (formerly BEIS)(2017), Road transport energy consumption at regional and local authority level, 2015) Baseline trajectory sees this efficiency increased to 3.15 TWh/bn vehicle-km by 2050. For the most ambitious (L4) scenario, the efficiency in 2050 is 1.34TWh/bn vehicle-km.
[1]Department for Energy Security & Net Zero (2018); Total final energy consumption at regional and local authority level
[2]Department for Energy Security & Net Zero (2019); Road transport energy consumption at regional and local authority level
[3]NTS9904; Average distance travelled by mode, region and Rural-Urban Classification: England, since 2002/03; Department for Transport
[4]National Travel Survey factsheets; Department for Transport
[5]https://www.gov.uk/government/statistics/2011-rural-urban-classification
[6]Department for Transport (2018) UK Aviation Forecasts 2017, Table 69 Carbon emissions by airport, baseline capacity, MtCO2
[7] Department for Transport, UK Aviation Forecasts 2017 p. 83
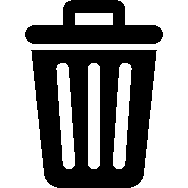
Waste
Increase in rates of recycling
- Metric: Increase in proportion of total waste directed towards recycling.
- Emissions sources affected:
- Open-loop
- Closed-loop
- Landfill
- Composting
- Combustion
- Wastewater
- Interventions:
1. 65% recycling, 10% landfill, 25% incineration by 2040; remaining constant to 2050.
2. 65% recycling, 10% landfill, 25% incineration achieved by 2035 remaining constant to 2050.
3. 65% recycling, 10% landfill, 25% incineration achieved by 2035, recycling rates increasing to 75% by 2050.
4. 65% recycling, 10% landfill, 25% incineration achieved by 2035, recycling rates increasing to 85% by 2050.
This lever interacts with reduction in volume of waste to define the total waste arisings and which waste stream they are captured in. Here, trajectories calculate the percentage recycling, landfill and "other" waste, applying these changes to the waste recorded in each category.
The "base case" is that the EU targets for 65% recycling are reached in 2035[1]; subsequent trajectories have different anticipated dates for reaching this. In Scenario 2, 65% recycling is met between 2045 and 2050. In Scenario 3, recycling increases steadily from 65% just after 2035 to 81% in 2050. In scenario 4, the recycling target is met earlier than 2035 and by 2050 85% all waste is recycled. The scenarios are applied to reported recycled and landfilled waste, as the change in the anticipated % waste recycled.
Reduction in volume of waste
- Metric: Reduction in volume of waste
- Emissions sources affected:
- Open-loop
- Closed-loop
- Landfill
- Composting
- Combustion
- Wastewater
- Interventions:
1. Total volume of waste is 124% of 2017 levels by 2040.
2. Total volume of waste is 108% of 2017 levels by 2040.
3. Total volume of waste is 85% of 2017 levels by 2040.
4. Total volume of waste is 61% of 2017 levels by 2040.
Total volume of waste arising is calculated by type (Household, Commercial & Industrial, Construction & Demolition) according to defined percentage changes in each. This total is summed for each five-year period. The change in this total each year is applied to all types of reported waste for the local authority.
By simplifying the trajectory, and applying the same reduction in wastage rates uniformly, a level of detail between different types of waste arisings has been lost. However, the original waste trajectories are similar.
[1] European Waste targets for 2035 https://www.letsrecycle.com/news/latest-news/eu-set-softer-targets-55-recycling-2025/
Icons made by https://www.flaticon.com/authors/monkik